Part 4: Humanized immune checkpoint mouse models for immuno-oncology studies
The immune system must maintain an intricate balance between the identification and elimination of “non-self” antigens and the suppression of any uncontrolled immune response that might attack “self” antigens.1 This delicate interplay is regulated by immune checkpoints (ICPs), a plethora of stimulatory and inhibitory molecules naturally expressed both on T cells and antigen-presenting cells. ICPs have been compared to accelerators and brakes, as they ensure the immune system is sufficiently engaged in its attack against exogenous microorganisms while avoiding excessive activation that can destroy healthy cells and tissues.2
Interestingly, ICPs are also found on the outer membrane of many tumor cells, allowing them to cleverly evade the host immune response.3 These findings have revolutionized cancer treatments, changing the way we view how cancer can be managed. Several preclinical studies have demonstrated that antibodies directed against key ICPs inhibit their function, enabling the elimination of certain tumor cells. For this reason, a number of co-stimulatory molecules and checkpoint inhibitors are used to treat certain types of cancers, offering patients a durable remission from diseases whose outcomes were once inevitably terminal.4–6
However, before transferring results from preclinical models to the bedside, clinical validation of the therapeutics is necessary: immuno-oncology compounds must be carefully assessed in preclinical ICP models to predict their efficacy before launching any human trial. The sad, well-known story of the CD28 superagonist antibody TGN1412 illustrates this principle well.7 Two key, interrelated aspects must be considered when choosing a humanized ICP model for drug discovery programs: i) the specific question(s) the research needs to address, and ii) the translatability and functionality of the model. With these two concepts in mind, we present specific examples of humanized ICP models currently used in drug development pipelines.
The preclinical humanized CD28 (hCD28) mouse model represents an excellent example of a model whose experimental design was driven by the need to accurately address specific biological questions. More particularly, this model was conceived to profile and assess the efficacy of immuno-oncology agents targeting the human CD28 in fully immunocompetent mice. The hCD28 model expresses a chimeric CD28 with a human extracellular domain, which confers the versatility to test any biologics targeting the extracellular domain of hCD28, and murine transmembrane and intracellular domains, which should optimize the anchoring of the protein to the membrane and its intracellular signaling. Yet, in a recent study, Tuosto and colleagues demonstrated that a single amino acid variant in the intracellular domain of CD28 seems to regulate differential signaling between the human CD28 and mouse CD28, resulting in an enhanced inflammatory response in humans.2,8 Because the hCD28 model features a murine intracellular domain, its use is recommended to run efficacy but not safety nor toxicity studies, as the results obtained using this model may underestimate the inflammatory responses induced upon hCD28 activation.
Another important point to consider when choosing a humanized ICP model for drug discovery studies is its translatability and functionality. That is why it is so important that the humanized model displays the same biological features (e.g., expression levels and regulation of the ICP to target, and the preservation of the humanized ICP-murine ligand interaction) of its murine counterpart, as exemplified by the humanized PD-1 (hPD-1) and CTLA-4 (hCTLA-4) mouse models. As shown in Figure 1, the expression pattern of hPD-1 in hPD-1 mice recapitulates the murine PD-1 (mPD1) well, making this model a powerful tool to evaluate human-specific PD-1 therapeutics such as pembrolizumab in vivo. A recent study has demonstrated that administering pembrolizumab induces anti-tumor response in hPD-1 mice inoculated with MC38 and GL261 tumor models.a,11
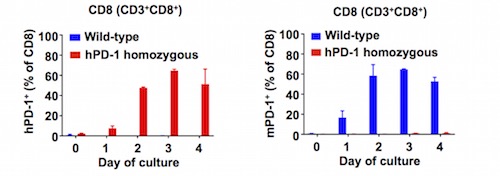
Figure 1) Human PD-1 (hPD‑1) expression pattern recapitulates mouse PD‑1 (mPD-1).
Similarly, humanized CTLA-4 (hCTLA-4) mice implanted subcutaneously with MC38 colorectal carcinoma were recently shown to achieve significantly increased life spans when treated with ipilimumab compared to both the control group and the one treated with murine anti-CTLA-4 (9H10) antibody (Figure 2 and unpublished data). Interestingly, these mice possess a chimeric CTLA-4 whose extracellular domain is humanized, enabling in vivo efficacy assessment and profiling of any biologics targeting the human CTLA-4.

Figure 2) In vivo anti-tumor activity is observed in response to ipilimumab in hCTLA‑4 mice.
Combination therapies and bispecific antibodies are being used more and more to treat certain types of cancers, as, over time, some tumors develop resistances to checkpoint inhibitors and/or co-stimulatory molecules, including CTLA-4. These limitations have pushed scientists to understand the biological mechanisms responsible for developing such resistances, and establish new therapeutic strategies using humanized ICP models.12
Along this line, researchers at Alligator Bioscience generated a bispecific antibody, ATOR-1015, targeting the human ICPs CTLA-4 and OX40. This compound was assessed in humanized OX-40 (hOX40) mice, where it enhanced immune activation in the tumor area, improved clinical efficacy, and reduced toxicity compared to last-generation CTLA-4 monotherapies. Interestingly, this treatment also induced a tumor-specific immunological memory, suggesting that the hOX40 model supports long-term studies. Experiments conducted in hOX40 mice revealed that ATOR-1015 acts synergistically with anti-PD-1/PD-L1 therapy. The data obtained in this study have allowed starting a first-in-human trial to treat patients with advanced and/or refractory solid malignancies.13
More recently, humanized ICP models have been also used to support and evaluate the efficacy of novel anticancer treatments versus renowned ones (Figure 3). For example, a group of researchers at Bristol-Myers Squibb demonstrated that tumor growth in humanized VISTA (hVISTA) mice bearing MC38 cells is reduced in animals treated with combination therapy (anti-VISTA and anti-PD-1) compared to those that received monotherapy (anti-PD-1). Johnston and colleagues found that VISTA binds and suppresses T cells at acidic pH such as that typically measured in tumor microenvironments. Pharmacokinetic experiments conducted on hVISTA mice treated with VISTA-blocking antibodies showed that acidic pH-selective antibodies accumulate preferentially within tumors and exhibit longer serum mean resistance time than non-pH-selective antibodies.14

Figure 3) Anti-tumor effects in response to anti-human VISTA + anti-mouse PD-1 treatment.
In another paper, recently published in Science, a team of researchers at the Geisel School of Medicine at Dartmouth and ImmuNext sheds some light on using hVISTA mice to perform efficacy studies not only in immune-oncology but also in autoimmune and immune-inflammation diseases. ElTanbouly, Zhao and colleagues demonstrated that VISTA is critical in maintaining T cell self-tolerance in vivo. The authors elegantly showed that human VISTA agonist antibodies increased the graft versus host disease (GVHD) survival in hVISTA mice, confirming the findings in wild-type mice treated with surrogates antibodies, and validating the efficacy of their antibodies targeting human VISTA.15
Humanized ICP models represent, therefore, key tools in our fight against cancers and immune-related diseases. The first syngeneic mouse models expressing humanized ICP were developed approximately a decade ago, and since then, they represent a valid alternative to assess biologics targeting human ICPs. Before that, anticancer therapies were tested in immunodeficient mice engrafted with human xenografts; however, since these models lack a functional immune system, they were not suitable to evaluate the efficacy and safety of such treatments.16 Instead, scientists turned to immunodeficient mice reconstituted with human immune system (HIS), but due to species-related differences, HIS models do not necessarily recapitulate the reciprocal interactions between tumor and associated immune and stromal cells, and so they may not be suitable to study therapies targeting such components.
Humanized ICP mice, on the other hand, can be engrafted with well-established mouse tumor lines, including MC38, CT26, and MB49,b while keeping the proper interaction between stroma, immune cells and tumor cells, and a functional immune system: they allow the readout of mouse, but not human, cell responses. Another main advantage of these models is that they do not require surrogates’ antibodies, which greatly reduces the complexities and challenges of translating preclinical efficacy data into the clinic.19
However, it is important to develop a line of sight to the clinic at the early steps of drug discovery projects, as this will help in selecting the most appropriate humanized ICP model, i.e., the one that best addresses the study's research aim(s) within the research constraints. The experimental design of the model is important, because using an inappropriate model for hazard identification may alter data interpretation.
a MC38 and GL261 are murine colon adenocarcinoma and glioma models, respectively.9,10
b MB49 and CT26 are murine bladder cancer and colon carcinoma models, respectively.17,18
See also:
Part 1: Toward a Better Effective Preclinical Model in Immuno-Oncology
Part 2: BRGSF, a New Immunodeficient Model for Immuno-Oncology Studies
Part 3: BRGSF-HIS, a New Human Immune System Mouse Model for Immuno-Oncology Studies
Part 5: HSA/hFcRn, a powerful model for your PK/PD studies
References:
- Pardoll DM. The blockade of immune checkpoints in cancer immunotherapy. Nat Rev Cancer. 2012;12(4):252-264. doi:10.1038/nrc3239
- Zang X. 2018 Nobel Prize in medicine awarded to cancer immunotherapy: Immune checkpoint blockade – A personal account. Genes Dis. 2018;5(4):302-303. doi:10.1016/j.gendis.2018.10.003
- Paluch C, Santos AM, Anzilotti C, Cornall RJ, Davis SJ. Immune Checkpoints as Therapeutic Targets in Autoimmunity. Front Immunol. 2018;9:2306. doi:10.3389/fimmu.2018.02306
- Darvin P, Toor SM, Sasidharan Nair V, Elkord E. Immune checkpoint inhibitors: recent progress and potential biomarkers. Exp Mol Med. 2018;50(12):1-11. doi:10.1038/s12276-018-0191-1
- Auslander N, Zhang G, Lee JS, et al. Robust prediction of response to immune checkpoint blockade therapy in metastatic melanoma. Nat Med. 2018;24(10):1545-1549. doi:10.1038/s41591-018-0157-9
- Xu F, Jin T, Zhu Y, Dai C. Immune checkpoint therapy in liver cancer. J Exp Clin Cancer Res. 2018;37(1):110. doi:10.1186/s13046-018-0777-4
- Attarwala H. TGN1412: From Discovery to Disaster. Journal of Young Pharmacists. 2010;2(3):332-336. doi:10.4103/0975-1483.66810
- Porciello N, Grazioli P, Campese AF, et al. A non-conserved amino acid variant regulates differential signalling between human and mouse CD28. Nat Commun. 2018;9(1):1080. doi:10.1038/s41467-018-03385-8
- McIntyre RE, Buczacki SJA, Arends MJ, Adams DJ. Mouse models of colorectal cancer as preclinical models. BioEssays. 2015;37(8):909-920. doi:10.1002/bies.201500032
- Szatmari T, Lumniczky K, Desaknai S, et al. Detailed characterization of the mouse glioma 261 tumor model for experimental glioblastoma therapy. Cancer Science. 2006;97(6):546-553. doi:10.1111/j.1349-7006.2006.00208.x
- Avrutskaya A, Sonego F, Hauser J, et al. Abstract LB-063: Validation of humanized PD-1 knock-in mice as an emerging model to evaluate human specific PD-1 therapeutics. In: Immunology. American Association for Cancer Research; 2019:LB-063-LB-063. doi:10.1158/1538-7445.AM2019-LB-063
- Wang J, Wu G, Manick B, et al. VSIG-3 as a ligand of VISTA inhibits human T-cell function. Immunology. 2019;156(1):74-85. doi:10.1111/imm.13001
- Kvarnhammar AM, Veitonmäki N, Hägerbrand K, et al. The CTLA-4 x OX40 bispecific antibody ATOR-1015 induces anti-tumor effects through tumor-directed immune activation. J Immunother Cancer. 2019;7(1):103. doi:10.1186/s40425-019-0570-8
- Johnston RJ, Su LJ, Pinckney J, et al. VISTA is an acidic pH-selective ligand for PSGL-1. Nature. 2019;574(7779):565-570. doi:10.1038/s41586-019-1674-5
- ElTanbouly MA, Zhao Y, Nowak E, et al. VISTA is a checkpoint regulator for naïve T cell quiescence and peripheral tolerance. Science. 2020;367(6475):eaay0524. doi:10.1126/science.aay0524
- Sanmamed MF, Chester C, Melero I, Kohrt H. Defining the optimal murine models to investigate immune checkpoint blockers and their combination with other immunotherapies. Annals of Oncology. 2016;27(7):1190-1198. doi:10.1093/annonc/mdw041
- Chan E, Patel A, Heston W, Larchian W. Mouse orthotopic models for bladder cancer research. BJU International. 2009;104(9):1286-1291. doi:10.1111/j.1464-410X.2009.08577.x
- Lechner MG, Karimi SS, Barry-Holson K, et al. Immunogenicity of Murine Solid Tumor Models as a Defining Feature of In Vivo Behavior and Response to Immunotherapy. Journal of Immunotherapy. 2013;36(9):477-489. doi:10.1097/01.cji.0000436722.46675.4a
- Olson B, Li Y, Lin Y, Liu ET, Patnaik A. Mouse Models for Cancer Immunotherapy Research. Cancer Discov. 2018;8(11):1358-1365. doi:10.1158/2159-8290.CD-18-0044
Related products
Catalogue product
Customized product
Scientific excellence
From model design to experimental results
Tailor-made solutions adapted to scientific questionsRobust validation data on catalog models
Comprehensive dataset package
Generated with biopharma partners and in-houseCustomer care
Scientific follow-up and advice along the project
Collaborative approach for problem solving and development of innovative modelsEasy and fast access to models
Breeding facilities in US and Europe
Certified health status from professional breeders